Gautel, M. & Djinović-Carugo, K. The sarcomeric cytoskeleton: from molecules to motion. J. Exp. Biol. 219, 135–145 (2016).
Barrick, S. K. & Greenberg, M. J. Cardiac myosin contraction and mechanotransduction in health and disease. J. Biol. Chem. 297, 101297 (2021).
Lange, S., Pinotsis, N., Agarkova, I. & Ehler, E. The M-band: the underestimated part of the sarcomere. Biochim. Biophys. Acta Mol. Cell Res. 1867, 118440 (2020).
Al-Khayat, H. A., Kensler, R. W., Morris, E. P. & Squire, J. M. Three-dimensional structure of the M-region (bare zone) of vertebrate striated muscle myosin filaments by single-particle analysis. J. Mol. Biol. 403, 763–776 (2010).
Al-Khayat, H. A., Morris, E. P., Kensler, R. W. & Squire, J. M. Myosin filament 3D structure in mammalian cardiac muscle. J. Struct. Biol. 163, 117–126 (2008).
Caremani, M. et al. Low temperature traps myosin motors of mammalian muscle in a refractory state that prevents activation. J. Gen. Physiol. 151, 1272–1286 (2019).
Zoghbi, M. E., Woodhead, J. L., Moss, R. L. & Craig, R. Three-dimensional structure of vertebrate cardiac muscle myosin filaments. Proc. Natl Acad. Sci. USA 105, 2386–2390 (2008).
Brunello, E. et al. Myosin filament-based regulation of the dynamics of contraction in heart muscle. Proc. Natl Acad. Sci. USA 117, 8177–8186 (2020).
Main, A., Fuller, W. & Baillie, G. S. Post-translational regulation of cardiac myosin binding protein-C: a graphical review. Cell. Signal. 76, 109788 (2020).
Ponnam, S., Sevrieva, I., Sun, Y.-B., Irving, M. & Kampourakis, T. Site-specific phosphorylation of myosin binding protein-C coordinates thin and thick filament activation in cardiac muscle. Proc. Natl Acad. Sci. USA 116, 15485–15494 (2019).
Freiburg, A. & Gautel, M. A molecular map of the interactions between titin and myosin-binding protein C. Eur. J. Biochem. 235, 317–323 (1996).
Ponnam, S. & Kampourakis, T. Microscale thermophoresis suggests a new model of regulation of cardiac myosin function via interaction with cardiac myosin binding protein-C. J. Biol. Chem. https://doi.org/10.1016/j.jbc.2021.101485 (2021).
Hanft, L. M., Fitzsimons, D. P., Hacker, T. A., Moss, R. L. & McDonald, K. S. Cardiac MyBP-C phosphorylation regulates the Frank–Starling relationship in murine hearts. J. Gen. Physiol. 153, e202012770 (2021).
Previs, M. J. et al. Phosphorylation and calcium antagonistically tune myosin-binding protein C’s structure and function. Proc. Natl Acad. Sci. USA 113, 3239–3244 (2016).
Bennett, P., Rees, M. & Gautel, M. The axial alignment of titin on the muscle thick filament supports its role as a molecular ruler. J. Mol. Biol. 432, 4815–4829 (2020).
Wang, Z. et al. The molecular basis for sarcomere organization in vertebrate skeletal muscle. Cell 184, 2135–2150 (2021).
Lemke, S. B. & Schnorrer, F. Mechanical forces during muscle development. Mech. Dev. 144, 92–101 (2017).
Tonino, P., Kiss, B., Gohlke, J., Smith, J. E. & Granzier, H. Fine mapping titin’s C-zone: matching cardiac myosin-binding protein C stripes with titin’s super-repeats. J. Mol. Cell. Cardiol. 133, 47–56 (2019).
Tskhovrebova, L. & Trinick, J. Making muscle elastic: the structural basis of myomesin stretching. PLoS Biol. 10, e1001264 (2012).
Eldemire, R., Tharp, C. A., Taylor, M. R. G., Sbaizero, O. & Mestroni, L. The sarcomeric spring protein titin: biophysical properties, molecular mechanisms, and genetic mutations associated with heart failure and cardiomyopathy. Curr. Cardiol. Rep. 23, 121 (2021).
Linke, W. A. & Hamdani, N. Gigantic business. Circ. Res. 114, 1052–1068 (2014).
Al-Khayat, H. A., Kensler, R. W., Squire, J. M., Marston, S. B. & Morris, E. P. Atomic model of the human cardiac muscle myosin filament. Proc. Natl Acad. Sci. USA 110, 318–323 (2013).
Heissler, S. M. & Sellers, J. R. Kinetic adaptations of myosins for their diverse cellular functions. Traffic 17, 839–859 (2016).
Tacke, S. et al. A streamlined workflow for automated cryo focused ion beam milling. J. Struct. Biol. 213, 107743 (2021).
Wang, Z. et al. Structures from intact myofibrils reveal mechanism of thin filament regulation through nebulin. Science 375, eabn1934 (2022).
Green, E. M. et al. A small-molecule inhibitor of sarcomere contractility suppresses hypertrophic cardiomyopathy in mice. Science 351, 617–621 (2016).
Alamo, L. et al. Lessons from a tarantula: new insights into muscle thick filament and myosin interacting-heads motif structure and function. Biophys. Rev. 9, 461–480 (2017).
Jumper, J. et al. Highly accurate protein structure prediction with AlphaFold. Nature 596, 583–589 (2021).
Woodhead, J. L. et al. Atomic model of a myosin filament in the relaxed state. Nature 436, 1195–1199 (2005).
Hooijman, P., Stewart, M. A. & Cooke, R. A new state of cardiac myosin with very slow ATP turnover: a potential cardioprotective mechanism in the heart. Biophys. J. 100, 1969–1976 (2011).
Yang, S. et al. Cryo-EM structure of the inhibited (10S) form of myosin II. Nature 588, 521–525 (2020).
Bennett, P., Craig, R., Starr, R. & Offer, G. The ultrastructural location of C-protein, X-protein and H-protein in rabbit muscle. J. Muscle Res. Cell Motil. 7, 550–567 (1986).
Offer, G., Moos, C. & Starr, R. A new protein of the thick filaments of vertebrate skeletal myofibrils: extraction, purification and characterization. J. Mol. Biol. 74, 653–676 (1973).
McNamara, J. W. et al. Ablation of cardiac myosin binding protein-C disrupts the super-relaxed state of myosin in murine cardiomyocytes. J. Mol. Cell. Cardiol. 94, 65–71 (2016).
Alamo, L. et al. Three-dimensional reconstruction of tarantula myosin filaments suggests how phosphorylation may regulate myosin activity. J. Mol. Biol. 384, 780–797 (2008).
Daneshparvar, N. et al. CryoEM structure of Drosophila flight muscle thick filaments at 7 Å resolution. Life Sci. Alliance 3, e202000823 (2020).
Hu, Z., Taylor, D. W., Reedy, M. K., Edwards, R. J. & Taylor, K. A. Structure of myosin filaments from relaxed Lethocerus flight muscle by cryo-EM at 6 Å resolution. Sci. Adv. 2, e1600058 (2016).
Rahmani, H. et al. The myosin II coiled-coil domain atomic structure in its native environment. Proc. Natl Acad. Sci. USA 118, e2024151118 (2021).
Irving, M. Regulation of contraction by the thick filaments in skeletal muscle. Biophys. J. 113, 2579–2594 (2017).
Obermann, W. M. et al. The structure of the sarcomeric M band: localization of defined domains of myomesin, M-protein, and the 250-kD carboxy-terminal region of titin by immunoelectron microscopy. J. Cell Biol. 134, 1441–1453 (1996).
Granzier, H. L. & Labeit, S. The giant protein titin. Circ. Res. 94, 284–295 (2004).
Bogomolovas, J. et al. Titin kinase ubiquitination aligns autophagy receptors with mechanical signals in the sarcomere. EMBO Rep. 22, e48018 (2021).
Puchner, E. M. et al. Mechanoenzymatics of titin kinase. Proc. Natl Acad. Sci. USA 105, 13385–13390 (2008).
Charton, K. et al. CAPN3-mediated processing of C-terminal titin replaced by pathological cleavage in titinopathy. Hum. Mol. Genet. 24, 3718–3731 (2015).
Fukuzawa, A. et al. Interactions with titin and myomesin target obscurin and obscurin-like 1 to the M-band – implications for hereditary myopathies. J. Cell Sci. 121, 1841–1851 (2008).
Lange, S. et al. Dimerisation of myomesin: implications for the structure of the sarcomeric M-band. J. Mol. Biol. 345, 289–298 (2005).
Hornemann, T. et al. Muscle-type creatine kinase interacts with central domains of the M-band proteins myomesin and M-protein. J. Mol. Biol. 332, 877–887 (2003).
Pernigo, S. et al. Binding of myomesin to obscurin-like-1 at the muscle M-band provides a strategy for isoform-specific mechanical protection. Structure 25, 107–120 (2017).
Luther, P. K. et al. Direct visualization of myosin-binding protein C bridging myosin and actin filaments in intact muscle. Proc. Natl Acad. Sci. USA 108, 11423–11428 (2011).
Risi, C. M. et al. Cryo-electron microscopy reveals cardiac myosin binding protein-C M-domain interactions with the thin filament. J. Mol. Biol. 434, 167879 (2022).
Tskhovrebova, L. & Trinick, J. Titin: properties and family relationships. Nat. Rev. Mol. Cell Biol. 4, 679–689 (2003).
Hagen, W. J. H., Wan, W. & Briggs, J. A. G. Implementation of a cryo-electron tomography tilt-scheme optimized for high resolution subtomogram averaging. J. Struct. Biol. 197, 191–198 (2017).
Mastronarde, D. N. SerialEM: a program for automated tilt series acquisition on Tecnai microscopes using prediction of specimen position. Microsc. Microanal. 9, 1182–1183 (2003).
Tegunov, D. & Cramer, P. Real-time cryo-electron microscopy data preprocessing with Warp. Nat. Methods 16, 1146–1152 (2019).
Kremer, J. R., Mastronarde, D. N. & McIntosh, J. R. Computer visualization of three-dimensional image data using IMOD. J. Struct. Biol. 116, 71–76 (1996).
Wagner, T. et al. SPHIRE-crYOLO is a fast and accurate fully automated particle picker for cryo-EM. Commun. Biol. 2, 218 (2019).
Schöenfeld, F., Stabrin, M., Shaikh, T. R., Wagner, T. & Raunser, S. Accelerated 2D classification with ISAC using GPUs. Front. Mol. Biosci. 9, 919994 (2022).
Moriya, T. et al. High-resolution single particle analysis from electron cryo-microscopy images using SPHIRE. J. Vis. Exp. https://doi.org/10.3791/55448 (2017).
Yang, Z., Fang, J., Chittuluru, J., Asturias, F. J. & Penczek, P. A. Iterative stable alignment and clustering of 2D transmission electron microscope images. Structure 20, 237–247 (2012).
Bharat, T. A. M. & Scheres, S. H. W. Resolving macromolecular structures from electron cryo-tomography data using subtomogram averaging in RELION. Nat. Protoc. 11, 2054–2065 (2016).
He, S. & Scheres, S. H. W. Helical reconstruction in RELION. J. Struct. Biol. 198, 163–176 (2017).
Pettersen, E. F. et al. UCSF ChimeraX: structure visualization for researchers, educators, and developers. Protein Sci. 30, 70–82 (2021).
Yamada, Y., Namba, K. & Fujii, T. Cardiac muscle thin filament structures reveal calcium regulatory mechanism. Nat. Commun. 11, 153 (2020).
Kidmose, R. T. et al. Namdinator – automatic molecular dynamics flexible fitting of structural models into cryo-EM and crystallography experimental maps. IUCrJ 6, 526–531 (2019).
Ermel, U. H., Arghittu, S. M. & Frangakis, A. S. ArtiaX: an electron tomography toolbox for the interactive handling of sub-tomograms in UCSF ChimeraX. Protein Sci. 31, e4472 (2022).
Kaur, S. et al. Local computational methods to improve the interpretability and analysis of cryo-EM maps. Nat. Commun. 12, 1240 (2021).
Buchholz, T.-O. et al. Content-aware image restoration for electron microscopy. Methods Cell Biol. 152, 277–289 (2019).
Makovetsky, R., Piche, N. & Marsh, M. Dragonfly as a platform for easy image-based deep learning applications. Microsc. Microanal. 24, 532–533 (2018).
Rees, M. et al. Making sense of missense variants in TTN-related congenital myopathies. Acta Neuropathol. 141, 431–453 (2021).
Wright, K. Antibodies a Laboratory Manual. Biochem. Educ. 17, 220 (1989).
Dutta, D., Nguyen, V., Campbell, K. S., Padrón, R. & Craig, R. Cryo-EM structure of the human cardiac myosin filament. Nature https://doi.org/10.1038/s41586-023-06691-4 (2023).
Huang, X. et al. Cryo-electron tomography of intact cardiac muscle reveals myosin binding protein-C linking myosin and actin filaments. J. Muscle Res. Cell Motil. 44, 165–178(2023).
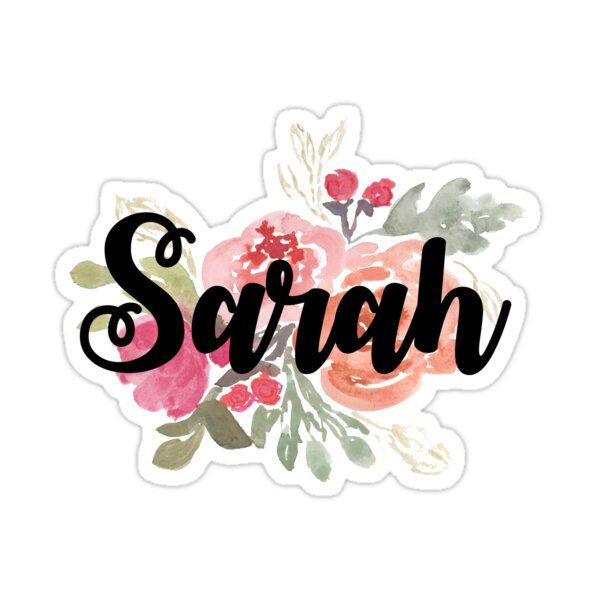
Sarah Carter is a health and wellness expert residing in the UK. With a background in healthcare, she offers evidence-based advice on fitness, nutrition, and mental well-being, promoting healthier living for readers.